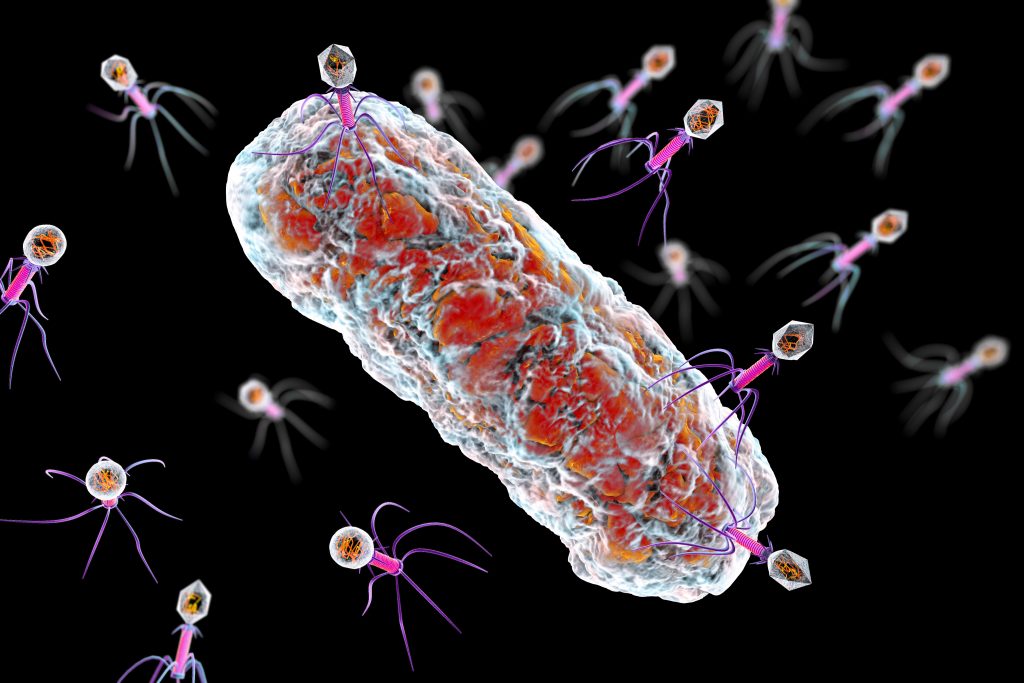
A 15-year-old patient with cystic fibrosis and a chronic antibiotic-resistant Mycobacterial infection was treated with a three-phage cocktail.
When Graham Hatfull, PhD, a professor at the University of Pittsburgh specializing in phage biology and James Soothill, MD, a microbiologist at Great Ormond Street Hospital for Children (GOSH) in London, met over 20 years ago at a phage biology meeting in Tbilisi, Georgia, they could not have predicted that they would one day collaborate to save the life of a teenage girl with a Mycobacterium infection. But, when a 15-year-old cystic fibrosis patient with a double lung transplant at GOSH acquired a disseminated Mycobacterium abscessus infection, Soothill knew exactly who to call to find phages that infect Mycobacterium.
The result of this collaborative effort is the first use of genetically engineered bacteriophages to treat a widespread Mycobacterial infection—which is notoriously difficult to treat with antibiotics—in a human patient. The case study is published today in Nature Medicine in a paper titled, “Engineered bacteriophages for treatment of a patient with a disseminated drug-resistant Mycobacterium abscessus.”
“My patient is doing well but we haven’t yet cured her of her infection,” notes Helen Spencer, MD, respiratory pediatrician at GOSH and senior author on the paper. “She is making steady progress and we hope that the infection will eventually clear.” The clinical improvement includes sternal wound closure, improved liver function (the hazardous infection in the liver was cleared in about 6 weeks), and substantial resolution of infected skin nodules. The nodules are resolving, but that process is occurring more slowly and is not yet complete.
This is a “clear example of the potential of bacteriophage therapy when phage biologists collaborate with clinicians within a careful but permissive regulatory environment,” notes Martha Clokie, PhD, professor of microbiology at the University of Leicester. “The data show that the phages were able to clear a complicated and multidrug-resistant bacterium when there were no other options left.”
Phage finding
“Finding the phages for these strains was difficult,” says Hatfull, adding that it was a real tour de force to get to the point of doing the therapy. What made it possible, according to Clokie, “was Graham’s long history of working with such a difficult bacterial group.”

Indeed, the Hatfull lab has one of the largest banks of phages in the country with a collection of 15,000 individual phage isolates and DNA sequences for roughly a fifth of them. This large bank is due, in large part, to the work of the participants of the SEA-PHAGES (Science Education Alliance-Phage Hunters Advancing Genomics and Evolutionary Science) program that is jointly managed by Hatfull’s group and the Howard Hughes Medical Institute’s Science Education division. This discovery-based undergraduate research course has resulted in large-scale phage collection and data collection.
“We used all of the information we have to try to find the phages that infect the one particular strain of Mycobacterium abscessus isolated from the patient,” notes Hatfull, telling GEN that they “got lucky” to find three phages that could be included in the cocktail. Hatfull lab members Rebekah M. Dedrick, PhD, and Carlos A. Guerrero-Bustamante, were deeply involved in the work to find the phages and were present in London when the phage cocktail was administered to the patient.

The first phage that they identified, named “Muddy”, killed the strain of Mycobacterium abscessus isolated from the patient very efficiently, with no modifications necessary. However, the second and third phages (named ZoeJ and BPs) were genetically engineered using technology that the Hatfull lab has developed over the last decade. The researchers were able to delete the key protein that switches the phages from their lytic (killing) state to their lysogenic (dormant stage marked by the integration of the phage genome into the bacterial chromosome) state. This genetic deletion locked the phages into their lytic mode and created more efficient bacterial killers. For the phage BPs, the researchers went a step further and screened the phages to isolate a mutant that was able to infect the particular isolate from the patient.
Their method of engineering, adds Hatfull, allowed them to precisely delete the genes without leaving scars or additional material. This was important because the phages are not considered recombinant, because no new DNA remained in the phage genome. The non-GMO status resulted in a simpler regulatory landscape. After months of work, the cocktail was administered intravenously and topically to the infected skin lesions.
Although there is nothing critical about the number three, per se, it was chosen because of the potential of the emergence of resistance—a concern that would result in the eventual failure of the treatment. To this end, Hatfull notes, that “three was a number that gave some confidence that we wouldn’t see resistance. The more phages, the more effectively you can counter that concern.” The team continually monitors the isolates from the patient for resistance, and, thankfully, the bacteria retain sensitivity. Hatfull adds that they have recently isolated a fourth phage that they can add to the cocktail to add more pressure onto the bacteria. The goal, Hatfull says, is to “help the phage do what they are doing as best they can.”
“They deserve a lot of credit,” adds Paul Hyman, PhD, associate professor of biology at Ashland University. “This was not a one-dose and done. They did a lot of monitoring, controls, tracking of phage in the patient’s system. It was a very well-organized study.”
Collaboration continues to be key
This published case study is one of a handful of cases that use intravenous phage therapy for systemic infections. One of the authors on this paper, Robert (Chip) Schooley, MD, professor of medicine and chief of the division of infectious diseases at the University of California, San Diego School of Medicine, was involved in the first of this type of cases, the pioneering and now well-known case of Tom Patterson’s recovery from an infection with a highly drug-resistant strain of Acinetobacter baumannii.
One of the reasons for excitement over this particular case is the bacteria at the center of it. Although Mycobacterium abscessus, a non-tuberculosis Mycobacteria (NTM), its similarity to the cause of tuberculosis, which is notoriously antibiotic resistant and kills 1.7 million people a year, makes the success of this approach in a Mycobacterium incredibly exciting. It is a proof of principle of using phage approaches in Mycobacteria that have long been thought to be recalcitrant to phage therapy because they live in macrophages and granulomatous structures.
Spencer notes, that “one has to be careful to draw any definite conclusion from an individual clinical case, but hopefully this will encourage further research into phage therapy for other antibiotic-resistant bacteria.”
This work “demonstrates a real need to have closer mapping of clinical need to phage research,” asserts Clokie, adding that “it is collaborations such as the one described in this paper where real progress can be made in developing this technology and allowing it to be more widely used.” Spencer adds that, “we know that antimicrobial resistance is a huge global concern. Collaboration is going to be critically important between health professionals, governments, and the pharmaceutical industry to tackle this increasingly important problem.”
source : www.genengnews.com